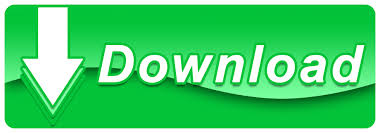
To address this need, we developed a mathematical model that describes the functional relationship between the abundance of the receptor protein and the main features of a biosensor response. (13−15) However, despite the experimental evidence, there is no formal theory that allows the design of customized biosensors in a systematic and predictable manner. Previous studies have reported that modulations in the receptor protein abundance either at transcriptional or post-transcriptional levels can be used to induce modifications in key biosensor parameters such as activation threshold, sensitivity, and dynamic range. The simplicity of this method, which allows specific modifications with minimal genetic manipulation, makes it suitable for designing synthetic bacterial sensors. Although different methods for tuning biosensor responses have been explored, (9−12) the simplest strategy is based on modulation of the abundance of the first component, i.e., the receptor protein. The first component of a TCB is a receptor protein that can bind an external signal and subsequently trigger the expression of the second TCB component, e.g., a fluorescent reporter protein. (8) More specifically, TCB signal detection relies on the interaction between the two components that is mediated by the external signal. In the present study, we focused on the architecture of the two-component bacterial biosensors (TCBs), which are the most abundant biosensors in prokaryotic organisms and which act as major signal transduction devices for sensing and responding to different environmental conditions. The good agreement between theoretical designs and experimental results indicates that modulation of receptor protein abundance allows optimization of biosensor designs with minimal genetic engineering. To experimentally validate the model and its predictions, a library of biosensors was constructed.

The defined mathematical framework allows the design of the genetic architecture of a two-component biosensor that can perform as required with minimal genetic engineering. We developed a mathematical model that describes the functional relationship between receptor abundance and activation threshold, sensitivity, dynamic range, and operating range. In this study, we explored the customizability of two-component bacterial biosensors by modulating the main biosensor component, i.e., the receptor protein. It is therefore often necessary to tune natural systems to meet the demands of specific applications in a predictable manner. However, biosensors rely not only on input detection but also on an adequate response range. Many studies have been devoted to the engineering of cellular biosensors by exploiting intrinsic natural sensors.
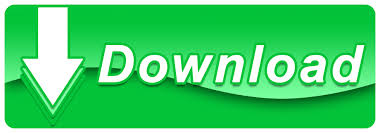